The building sector presents one of the main energy consumers in Europe [1]. In the global scope, the building sector accounts for 32% of the final energy consumption and 19% of greenhouse gas emissions [2]. A more recent study states that 16% of carbon dioxide (CO2) comes solely from buildings, where 11% is from residential and 5.9% from non-residential buildings, respectively [3]. Therefore, the importance of reducing energy consumption and the environmental impact of the building sector has also been pointed out by the Energy Performance of Buildings Directives (EPBD) [4] and in the revised EPBD [5] which brings forth the importance of existing buildings and energy-related retrofits. However, while research on improving the building energy performance of existing buildings and its implementations is well established in more developed countries [6], in developing countries such as Kosovo the uptake of energy efficiency measures (EEM) determined by regulations is still in the infantry. The issue with the low implementation of building performance regulations or non-compliance was also discussed in a study for developing countries [7] and in another study for both developing and developed countries [8]. Furthermore, the testing of standards and regulations has been a target of many research studies, where they are tested, or their performance is compared to simulations using different simulation tools. For instance, in research [9] they use the MEST EN ISO 13790 standard adopted by Montenegro for building energy performance, to compare the standard with RETScreen simulations for a hospital building. However, there is a lack of research studies evaluating the impact of the enacted regulations in Kosovo such as Regulation MESP-04/2018-RR [10] for minimum requirements for the energy performance of buildings, MESP -02/2018-RR [11] for the calculation methodology for the integrated energy performance of buildings and the heat demand. Furthermore, the motivation of this study is the issue of the high rate of non-compliance of building stakeholders to implement the regulations in practice therefore there is a need to emphasize and promote the impact of such measures.
The building energy performance can be increased either by implementing active measures e.g., by improving HVAC systems, or by implementing passive measures such as building retrofits [12]. The active measures were evaluated in a study conducted in Italy and Egypt [13] where different HVAC solutions are analyzed including district heating, heat pumps for cooling and heating, PV panels, and solar thermal systems. Their results show that the energy savings can reach up to 67% and the achieved period is 23 years for the case study in Egypt due to low energy cost. Additional studies were conducted for active EEMs in South India for detached family houses [14] and in China for residential buildings [15]. Their results showed that the primary energy consumption and environmental impact of direct electric heating systems is significantly higher than other heating systems with gas or heat pumps. The passive EEMs are addressed in a research for ten Italian cities [16] that emphasized the results of building retrofit are highly impacted by the climate zone, meaning that the payback period of the retrofit investments can vary from 3 years up to 84 years for different cities in Italy. Research [17] addresses the impact of different types of retrofits such as small, medium, and large retrofits and their results show a CO2 emission reduction of 18.56% with a payback period of 10.6 years. Many studies propose a combination of active and passive EEM to increase building energy performance such as in research [18] where they propose EEM for the building envelope, lighting, and appliances in different cities in Spain. In another similar study conducted in different cities in Portugal [19], different EEMs were considered such as envelope retrofits, lighting, appliances, PV panels, and heat pumps as HVAC solutions. The optimal solution according to this study is by using heat pumps. Therefore, from the literature review it is seen that it is also beneficial to evaluate the impact of active and passive EEM for Kosovo’s climate conditions on the building energy, economic and environmental impact.
In general, in residential buildings in Kosovo, only heating is used due to Kosovo’s climate, hence when accounting for HVAC energy consumption, it is mostly attributed to heating. According to [20], space heating in Kosovo accounts for 33% of the total energy demand. However, due to the lack of other heating sources such as renewable energy sources (RES) [21] or district heating in the majority of the country [22], one of the most used energies for heating is electricity. Using electricity that is produced by coal-based power plants is not an appropriate option for direct heating with electric heaters as it is not sustainable, and not environmentally [23], [24] or cost-friendly [15]. Research [20] also elaborated on means of evaluating the potential to reduce the heating demand on an urban scale for the city of Prishtina, Kosovo, by simulating the implementation of EEM to 23384 buildings of different typologies. Their results show that the heating demand can be reduced by 50% up to 68.5%, and the environmental impact can be reduced by 49.7% up to 68.3%. In comparison to a reference scenario, the remaining CO2 emissions reduce to 249.8 million kgCO2/year for the standard EEM scenario, and to 158.7 million kgCO2/year in the advanced EEM scenario, respectively. Lastly, the impact of different scenarios showed 65.2% up to 72.2% savings in space heat demand for apartment buildings solely. However, the impact of different HVAC systems on the total financial implications from the active and passive EEM in apartment buildings was not elaborated in previous studies and needs to be explored more. Furthermore, it is important to aim for higher energy efficiency of the buildings which will be addressed in this current study.
The building stock in Kosovo has had a major increase in recent years, with an emphasis on collective housing such as Apartment Blocks (hereinafter AB) which are mostly focused on urban areas. With the increment of the AB number, the building area and the residential units increased exponentially, which resulted in further increasing energy demands. Hence, the energy demand in general, and in the built environment specifically, needs to be addressed and evaluated to come up with interventions. For instance, in research [25] the increment of energy efficiency in residential buildings is elaborated for the Mediterranean Region by using design optimization. However, when the majority of the building stock is already existing, then retrofitting is needed.
Therefore, this research evaluates the impact of existing regulations on building energy performance for a reference building that is an AB built in 1990. The analysis emphasizes the impact of the implementation of the regulation on the heating demand, costs, and environmental impact for four different scenarios with varying EEM and HVAC systems. Furthermore, due to the lack of comprehensive reports on energy demand of the building sector in Kosovo, this research provides a thorough assembling and review of recent statistical data that is analyzed to provide insights on energy-related developments in Kosovo in general, and buildings specifically.
To analyze the impact of the implementation of the existing building energy performance regulations, these steps were used:
Mathematical models based on the Regulations MESP-04/2018-RR [10] and MESP-02/2018-RR [11] that are enacted in Kosovo for building energy performance based on the standard EN 13790.
Applying the mathematical models for a reference building as the case study to quantify the impact of implementing the energy efficiency measures and different HVAC solutions tested for four scenarios, in terms of energy demand, financial and environmental impact.
Comparison of the before and after conditions of the reference building.
The approach proposed by Regulation MESP-04/2018-RR [10] and Regulation MESP-02/2018-RR [11] to evaluate the heating demand was computed using the computer software Soft TAEK 2020 that is based on the expressions presented in [10].
The energy demand for space heating for each calculation period (month) is computed by:
(1)
where QL,H is the total heat losses, ηG,H is the utilization factor of heating gains and QG,H is the total heat gains in MJ. Total heat transfer (loss) for each month and each zone is given by the total heat transfer QT and the total heat transfer by ventilation QV in MJ. The equation is written as:
(2)
and the total gains for the building zone are:
(3)
where Qi is the sum of internal heat sources over the given period and Qs is the sum of solar heat sources in MJ. The total heat transfer by transmission is calculated for each month and each zone by:
(4)
where θi, θe,k are the internal and external temperatures respectively, t is the duration of the calculation period and f is the factor of conversion from Wh to MJ. The heat transfer coefficient HT,k through the building elements between the heated space and external air is computed by:
(5)
where Ai is the surface area of the building envelope, Ui is the thermal transmission coefficient, lk is the length of liner thermal bridge, and Ψk is the thermal conductivity. The units for these parameters are shown in the Nomenclature. The heat gains are calculated more specifically including the internal heat production Qi, the solar heat gain through transparent surfaces Qsun,t, and solar heat gain through opaque surfaces Qsun,nt. The equation is as follows:
(6)
The internal gains from internal sources are expressed by the heat production from occupants Qi,occ, internal heat production from appliances Qi,app and internal heat production from lighting Qi,li. The equation is written as:
(7)
And lastly, the heat transfer by ventilation for heating mode is calculated by:
(8)
where HV-heat is the ventilation heat loss coefficient, n is the number of days within a month. The environmental impact, namely the CO2 emission are calculated for each energy type for different scenarios using the fuel load Bfuel and the specific CO2 emission of the fuel:
(9)
The fuel load Bfuel is calculated based on the heat demand QN,H and energy source used for each scenario through the LHV lower heating value of fuel and the boiler efficiency, using:
(10)
This paper analyses a reference AB building of Type D [26] (Figure 1), that was built in 1990 and is located among 19 other similarly built residential units in Sunny Hill 2, Prishtina, Kosovo. Table 1 presents the characteristics of the reference building.
The AB reference building for the building energy performance analysis
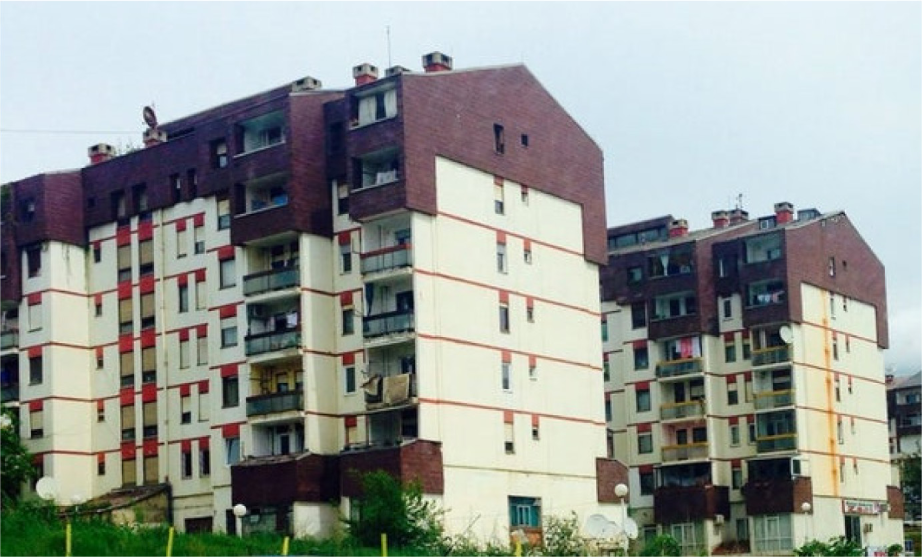
Reference building model characteristics Type D
Description |
Unit |
Description |
Unit |
---|---|---|---|
Floors |
P+5 |
Heated volume |
8,340.82 m3 |
Residential units per floor |
7 (seven) |
External walls area |
1,598.40 m2 |
The base area of the building |
710.64 m2 |
Windows and walls area |
529.20 m2 |
Gross volume of the building |
12,791.52 m3 |
Roof area |
710.64 m2 |
Heated area |
3,147.48 m2 |
|
|
The proposed EEM to improve the energy performance of the AB is retrofitting the building envelope, namely the external walls, windows and doors, floors, and roof. This includes wall insulation (k=0.04 W/mK), floor insulation (5 cm, k=0.04 W/mK) and change of windows with double panned glasses (U=1.60 W/m2K). Table 2 presents the existing and target values of the heat transfer coefficient (U, W/m2K) of the reference building model. The target values are based on Regulation MESP-04/2018-RR [10]. According to Table 2, the existing thermal coefficient values are at least two times higher than the target values.
Heat transfer coefficient U [W/m2K] for the building envelope for existing reference building and the target values according to [10]
Envelope type |
Existing value, W/m2K |
Target value, W/m2K [10] |
---|---|---|
External walls |
1.32 |
0.35 |
External doors and windows |
2.80 |
1.20 |
Floors |
0.62 |
0.50 |
Roof |
0.95 |
0.30 |
Four scenarios are proposed to identify the economic implication of different EEM measures applied for the same reference building model. The first scenario (S-01/NE-EB) presents the current state of the building using electric boilers for heating although the district heating system is installed but not connected to the heating grid. The second scenario (S-02/EE-EB) uses the same heating solution but also has EEM applied, the third one (S-03/EE-HP) has EEM and heat pump, and the fourth (S-04/EE-DH) has EEM and uses district heating. The proposed scenarios are:
Scenario 1: S-01/NE-EB: Existing heating solution using electric boilers and no EEM
Scenario 2: S-02/EE-EB: Heating with electric boilers after implementing EEM,
Scenario 3: S-03/EE-HP: Using air-water heat pump for heating after applying EEM,
Scenario 4: S-04/EE-DH: Using central district heating after implementing EEM.
The last three scenarios consider the same standard EEM, and the district heating is considered from “Termokos” Prishtina [27]. These scenarios are based on data retrieved from the construction years 1980-1999. To compute the primary energy cost for heating as well as the CO2 emissions, the data from Table 3 are used for the estimations.
Fuel costs and CO2 emissions for the market price for winter 2021/2022 [10]
Fuel type |
Cost, €c /kWh |
CO2 emission, kgco2/kWh |
---|---|---|
Wood |
0.035 |
0.031 |
Pellet |
0.051 |
0.034 |
Coal - Lignite |
0.021 |
0.378 |
Oil [28] |
0.124 |
0.030 |
Electricity [29] |
0.073 |
1.438 |
District heating [30] |
0.082 |
0.406 |
The following section shows a thorough presentation of the energy-related statistics in Kosovo, which were assembled and analysed to properly depict the impact of the building sector on the energy demand in recent years. Furthermore, it gives information on the building sector and building typologies in Kosovo.
According to the annual report of the Kosovo Regulatory Office (ERO) [31], Kosovo’s energy-generating capacities include 1,441.88 MW, including the energy from RES. Whereas the operating capacity only reaches 1,092.38 MW, where coal-based power plants (CPP) account for 87.88% and the rest 12.12% is from RES, namely hydro, wind, and solar systems. The distribution of these generating capacities is presented in Figure 2.
Since the primary electricity providers are two old CPP [21], and because low calorific value lignite is used as fuel, the amount of fossil fuel and consequently, the CO2 emissions from the energy sector in Kosovo are very high. Thus, using this electricity for direct heating purposes is a misuse of energy and should be avoided. However, due to the dearth of other heating alternatives, this is currently an ongoing concern from the energy and environmental perspective. Furthermore, the limited electricity production sources in Kosovo can barely keep up with the ever-growing electricity demand [32].
Participation in electricity generation in Kosovo 2020
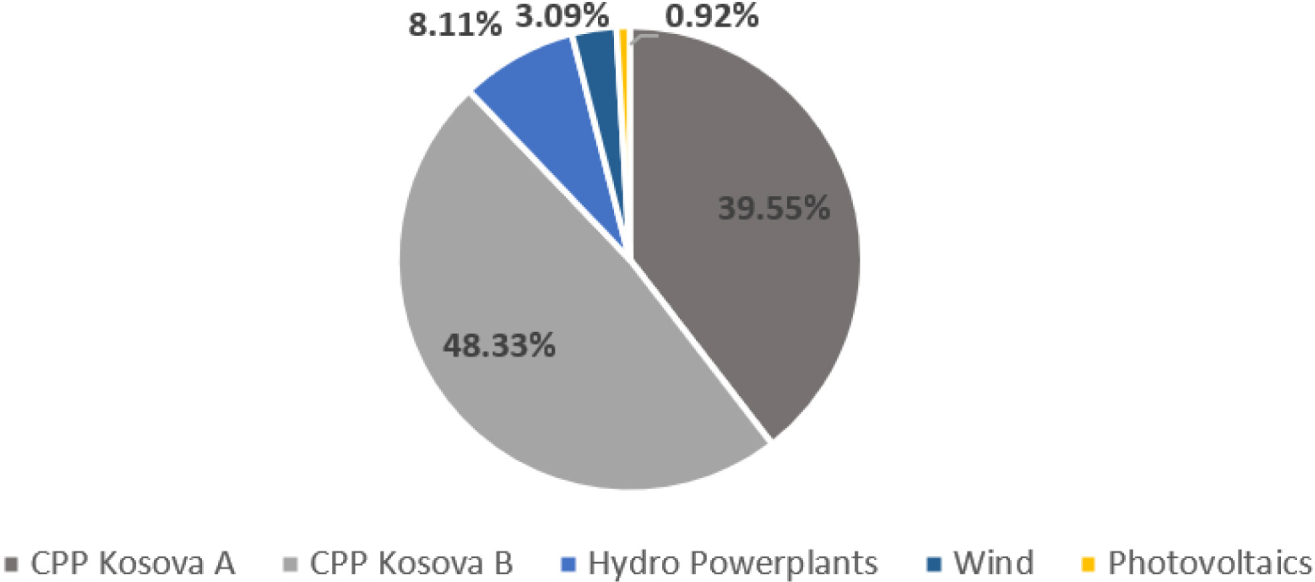
Compared to other Western Balkan countries, Kosovo is among the countries with low electricity costs, as shown in Figure 3. The prices were retrieved from the electricity costs for the Winter season 2021/2022, for Kosovo [33], Albania [34], Serbia [35], Montenegro [36], North Macedonia [37], and Bosnia and Herzegovina [38]. Considering that other neighboring countries apply different taxes to the end price of electricity; it is beneficial to illustrate the electricity price of Kosovo by comparing the same electricity demand in different countries for high and low tariffs. Therefore, a simple analysis was conducted as an example for illustration purposes to visualize the differences on the energy costs by assuming four scenarios (EC-01 up to 04) on different electricity demand depending on the high and low electricity tariffs. Table 4 shows the assumed scenarios, and the visualizations are depicted in Figure 4.
The proposed scenarios for monthly electricity consumption
Scenario |
High tariffs, kWh |
Low tariffs, kWh |
---|---|---|
Consumption Scenario EC-01 |
250 |
250 |
Consumption Scenario EC-02 |
500 |
500 |
Consumption Scenario EC-03 |
1000 |
500 |
Consumption Scenario EC-04 |
1500 |
500 |
Electricity costs calculated by adding the VAT for high and low tariffs (HT and LT) and value-added tax (VAT) for Western Balkan countries for the Winter season 2021/2022
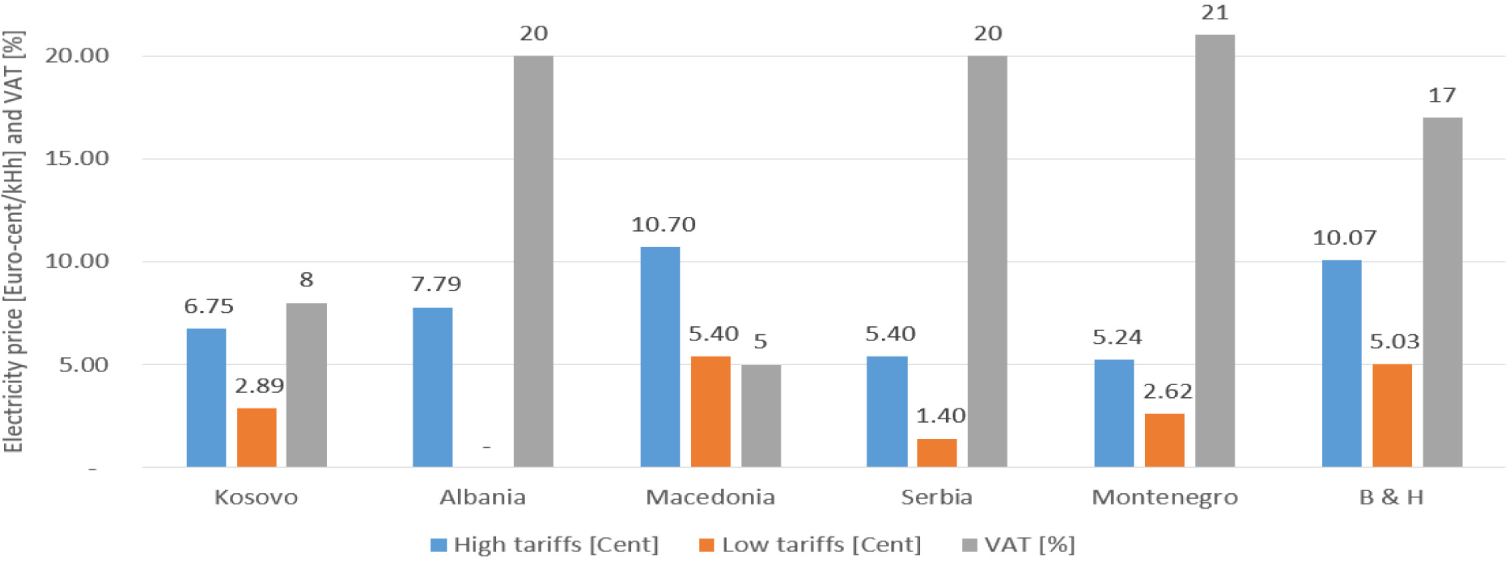
Figure 4 presents the depiction of the four assumed scenarios, and it shows that Kosovo had lower mean electricity costs when considering low and high tariffs and VAT, compared to the mean value of the electricity costs of five other Western Balkan Countries. The first scenario EC-01 has lower electricity costs for 32.28%, scenario EC-02 for 37.29%, scenario EC-03 for 36.08% and lastly scenario EC-04 for 37.90% compared to the mean value of the other 5 countries. These results are presented to visualize the differences in energy prices for the neighboring Balkan countries.
Electricity costs for neighbouring Western Balkan countries
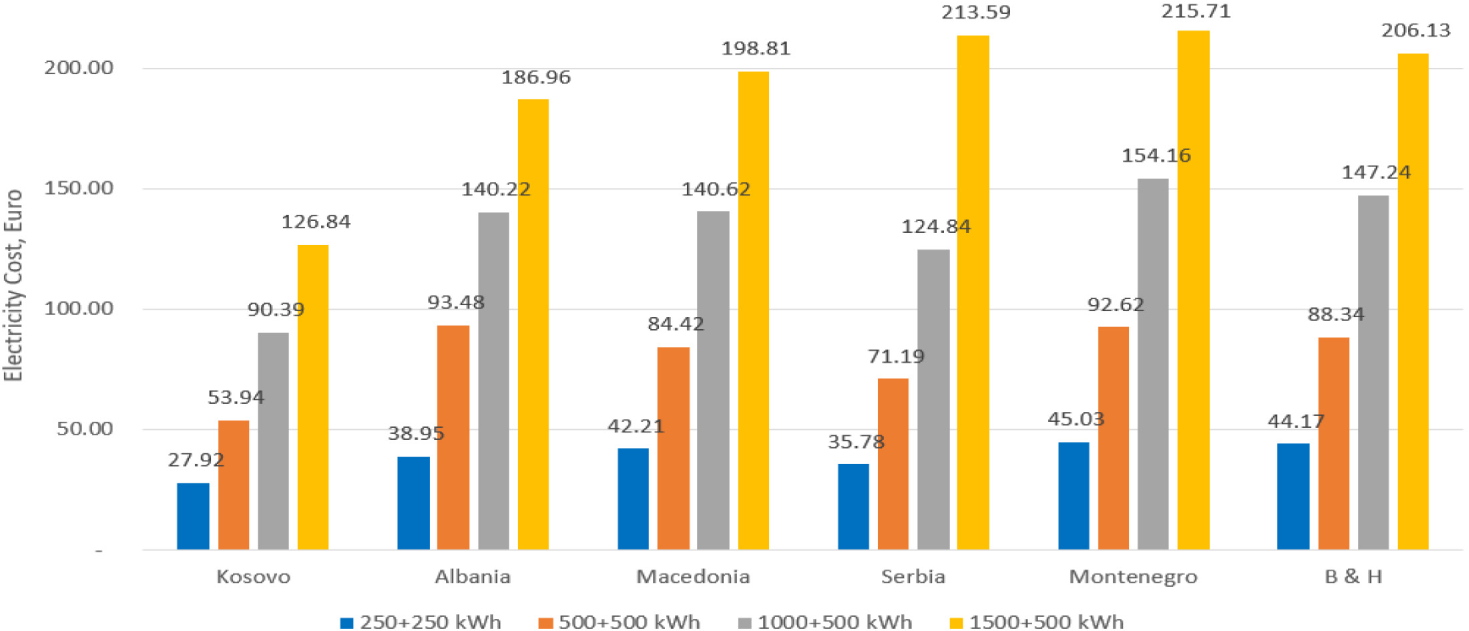
The electricity demand during the heating season (15October-15April) increases due to higher demand for heating in the domestic sector that uses electricity as the primary heating source. Figure 5 shows the comparison of electricity consumption between the domestic and commercial sectors in 2020 [32]. As a comparison, Figure 6 presents the electricity consumption for 2018, 2019 and 2020, where a drop of around 2% each year in electricity consumption is noted in the winter season due to the increasing distribution of district heating with cogeneration from CPP Kosova B power-plant.
Energy consumption for domestic and commercial use Electricity in 2020
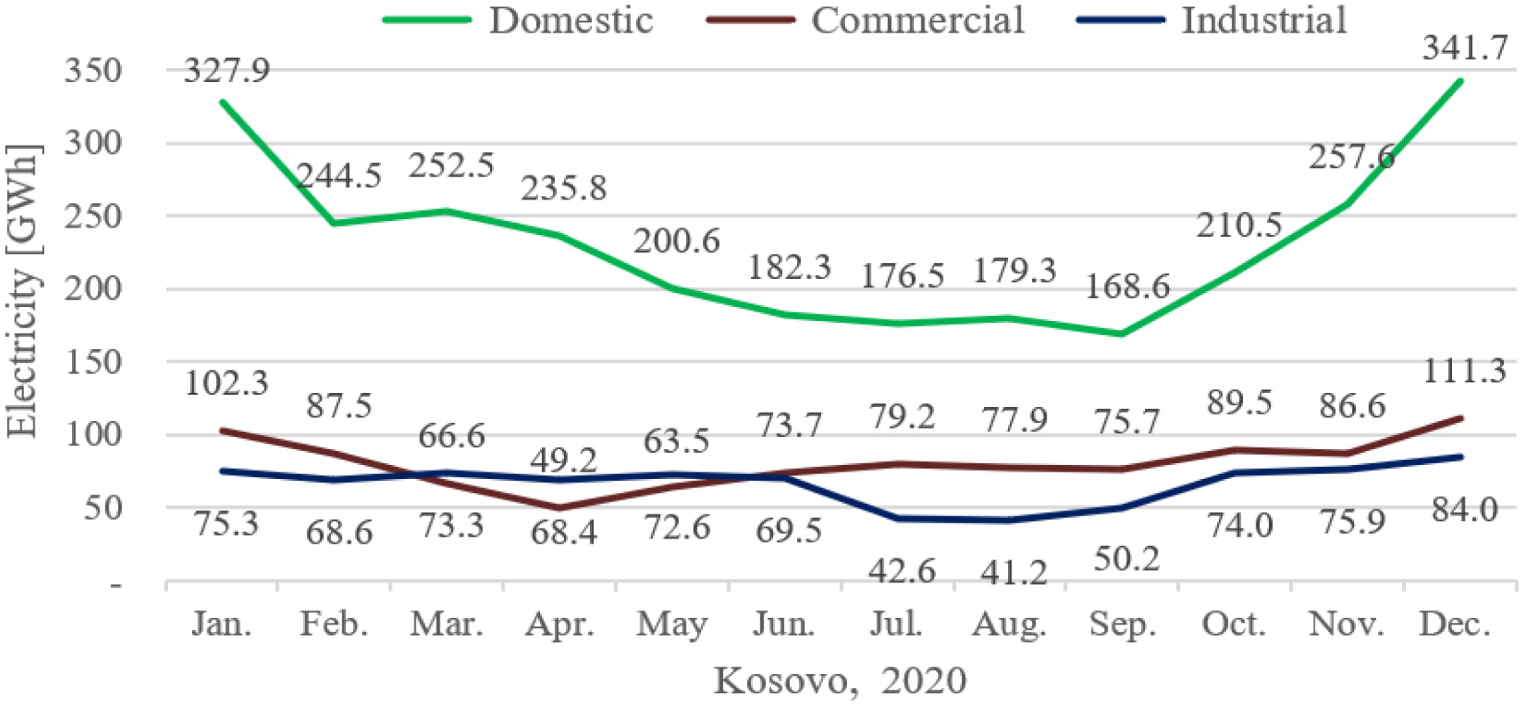
Comparison of the yearly domestic electricity consumption
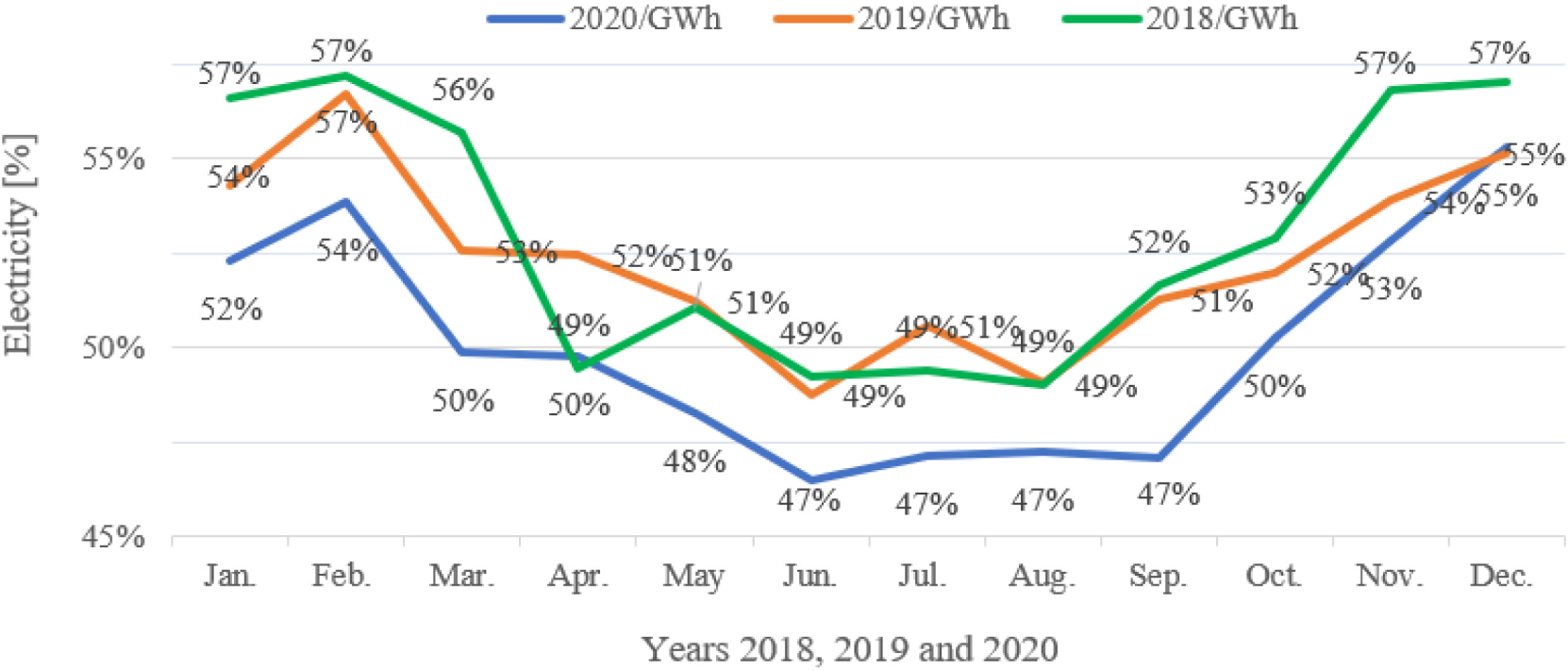
The electricity consumption in Prishtina municipality (Figure 7) which also presents the city with the highest concentration of buildings, accounts for 31.33% of the national energy consumption for domestic use [32]. Figure 8 compares the annual energy consumption based on the season and it shows that there is a significant increase in electricity consumption compared to 2017, by 4.16% and 10.20% in 2018 and 2019, respectively [32]. Additionally, the mean value of the monthly electricity consumption for these three years during the winter season shows that there is a 30.55% increase in the mean electricity consumption per month, which is mainly attributed to the use of electric boilers for heating purposes, but it also is affected by the domestic hot water demand, lighting, and other purposes.
Electricity consumption through the years of Prishtina district for domestic use
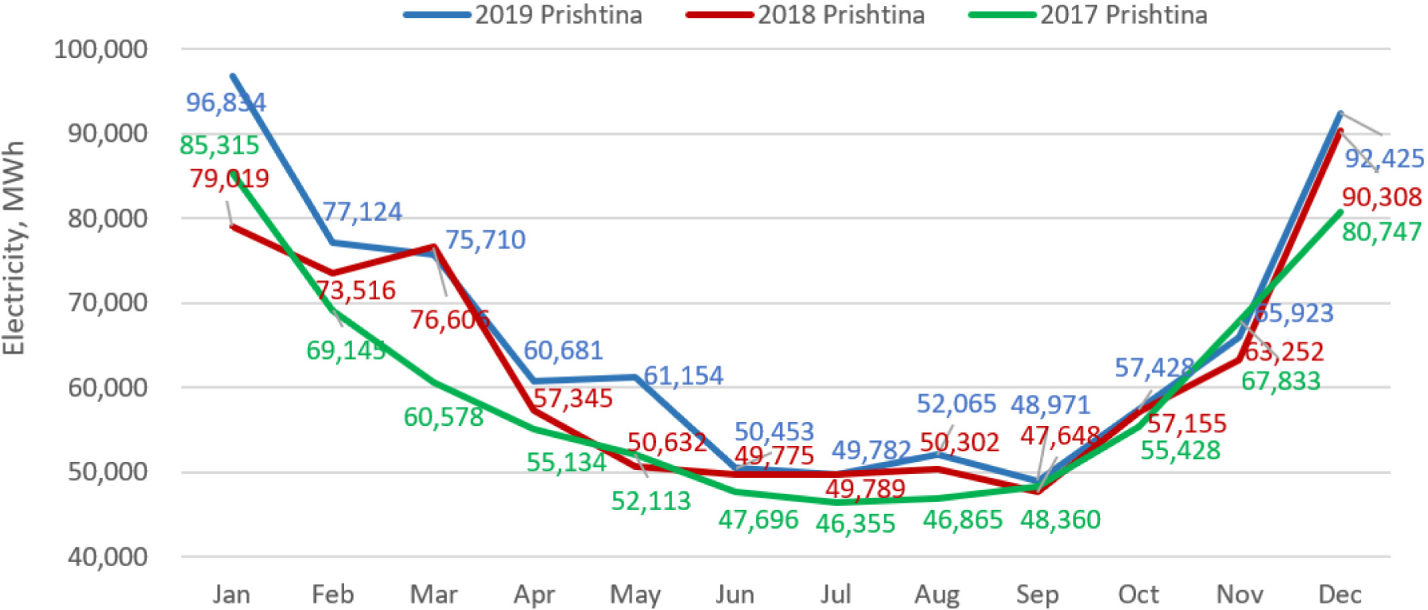
Monthly mean electricity consumption for Prishtina district
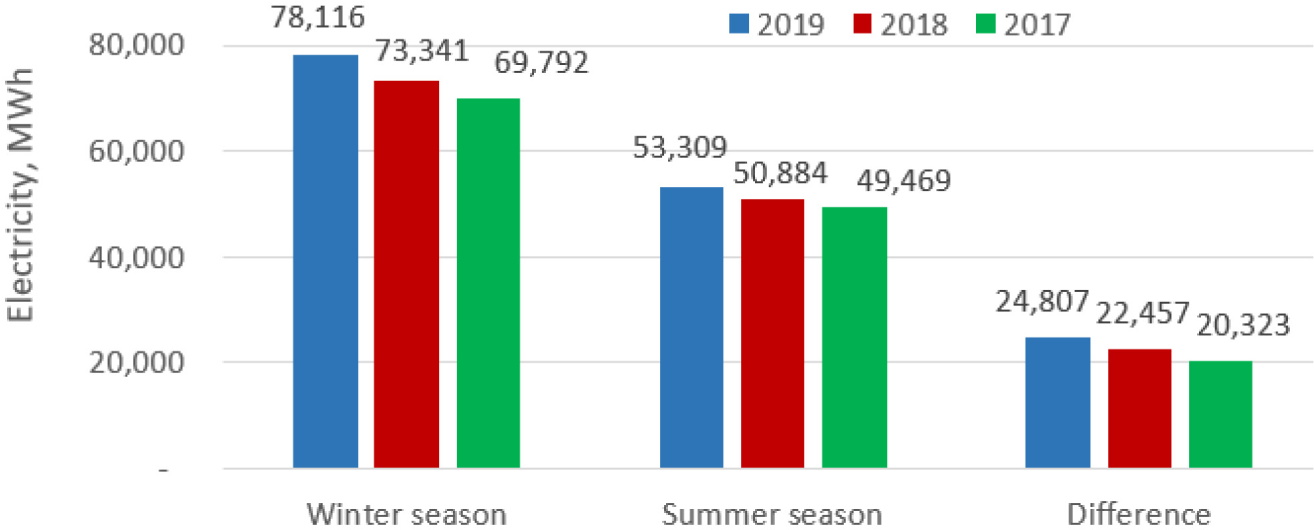
Another heating alternative that is highly used in Prishtina is district heating from cogeneration from the CPP Kosova B, which provides affordable prices compared to other heating solutions and has satisfactory services. Figure 9 presents the thermal energy consumption from Termokos [39] in Prishtina in 2020 [32], where it shows that the domestic and commercial thermal energy demand is very similar. Although the energy from the district heating is coal-based, in the future it can transition to more sustainable solutions to comply with the Zero carbon aim by 2050 for South East Europe as stated in [40].
Thermal energy consumption from Termokos in Prishtina in 2020
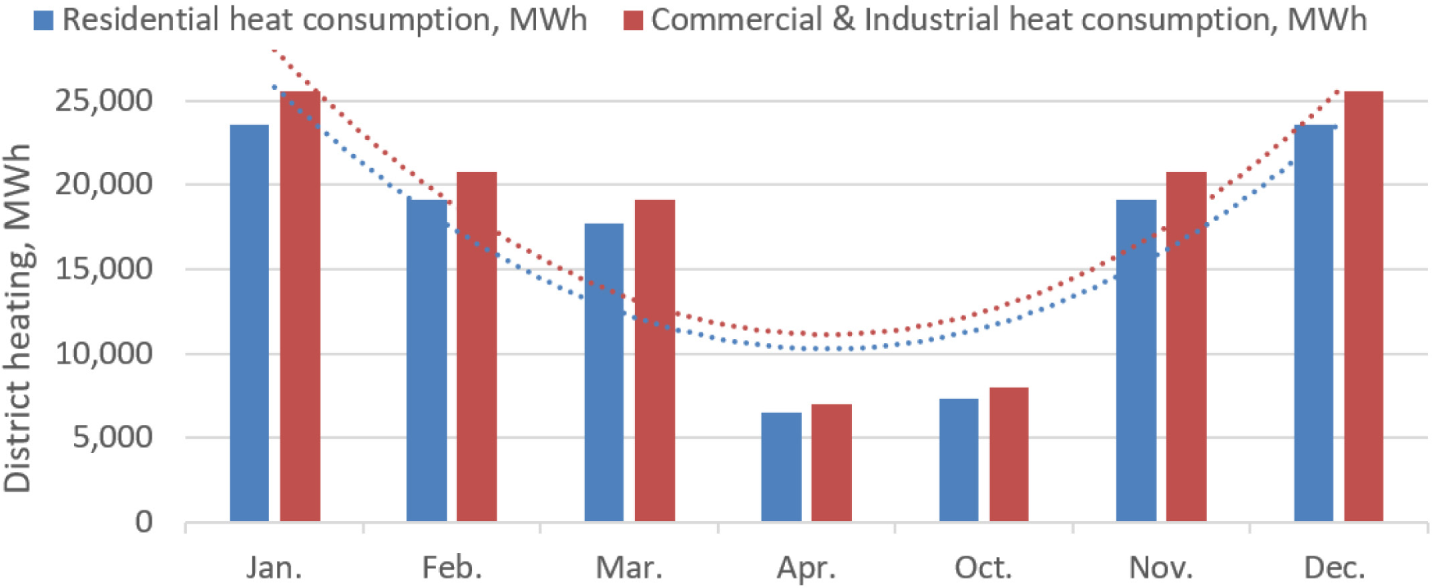
Although in Europe a substantial share of building stock is older than 50 years [1], in Kosovo the building stock is relatively new and the construction development is divided into four periods, namely 1960-1969, 1970-1979, 1980-1999 and 2000-2017 [26]. The number of buildings before 1960 was not considered in the statistics. A major surge in construction is evident in the years with turning historical points of Kosovo such as in the post-war period after 1999, as presented in Figure 10. This comes in contrast to Western countries where only a low rate of 1% growth of construction in the residential sector is reported in [41]. The statistics presented in [26] show that from 1960 up to 2017, the total number of registered buildings in Kosovo has been 247,680 units, the number of residential units is 412,883 and the gross total building area is 32,298,776 m2.
The share of residential units in the construction period 1960-2017 (the total number of buildings divided per the number of AB for each period)
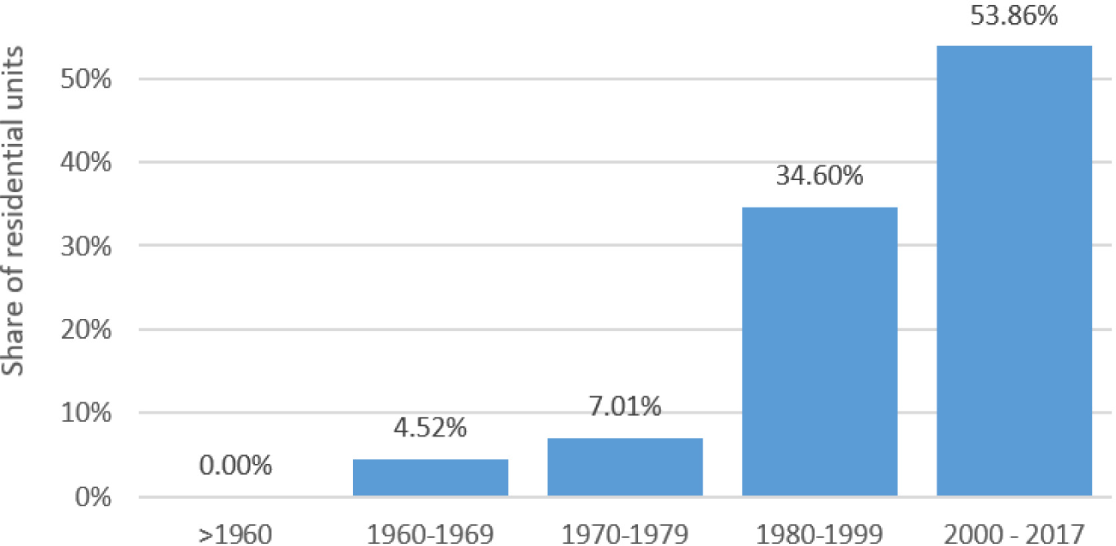
According to the same report [26], the built environment in Kosovo is characterized by four classes of buildings, namely: Type A: individual homes, Type B: terraced houses, Type C: low-rise block apartments, and Type D: high-rise block apartments (used as reference model in this study). The AB stock has had significant growth during the past few decades, especially during 1980-1999 and 2000-2017, as presented in Figure 11. Along these lines, in the first period, the constructed building area had an increase of 79.21%, as depicted in Figure 12. The AB presents a multi-story building with a large floor area consisting of three or more residential units. The AB account for a high share in the building stocks in Kosovo [26], specifically, 1.13% of the total number of buildings are AB, 28.32% of the total number of residential units, and 31.93% of the total gross building area. Therefore, they have high heating demand, but the actual main heating solution is electric boilers. The issue with heating supply in Kosovo is also asserted in the WBIF report [42], where the EU allocated additional grants to add central heating solutions in municipalities in Kosovo, as the existing central district heating in Kosovo (in Prishtina 140 MW, Gjakova 11 MW and Mitrovica 38MW) only cover 3-5% of heating demand on the national level [42].
The number of AB for 1960-2017
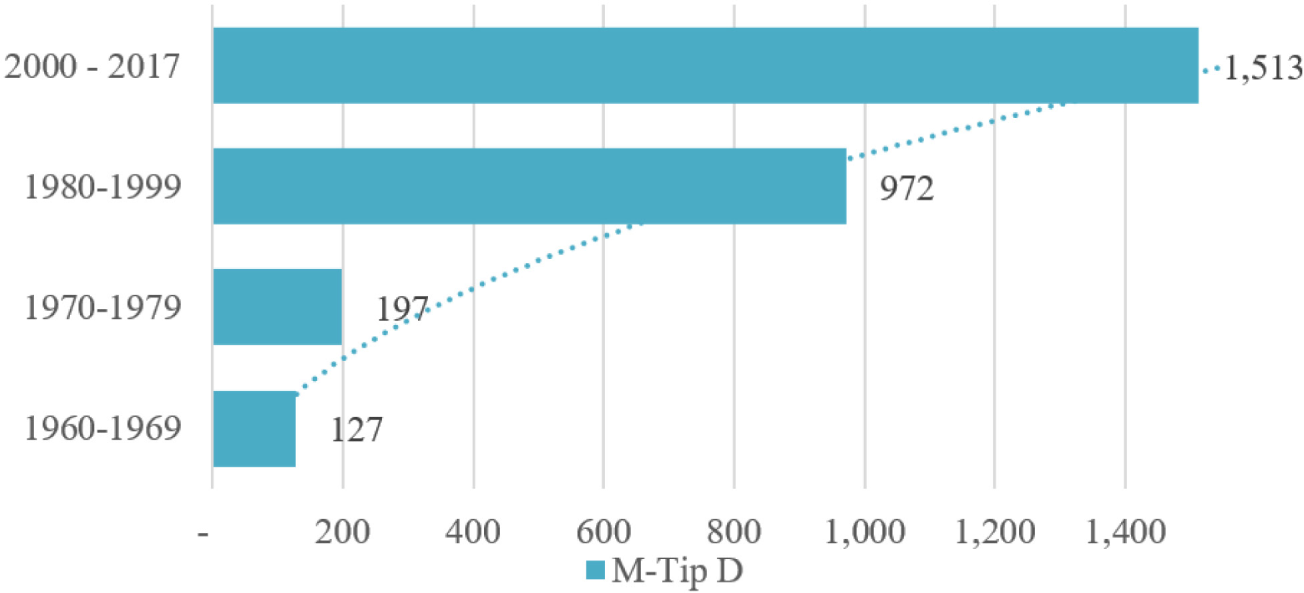
The amount of AB area for 1960-2017
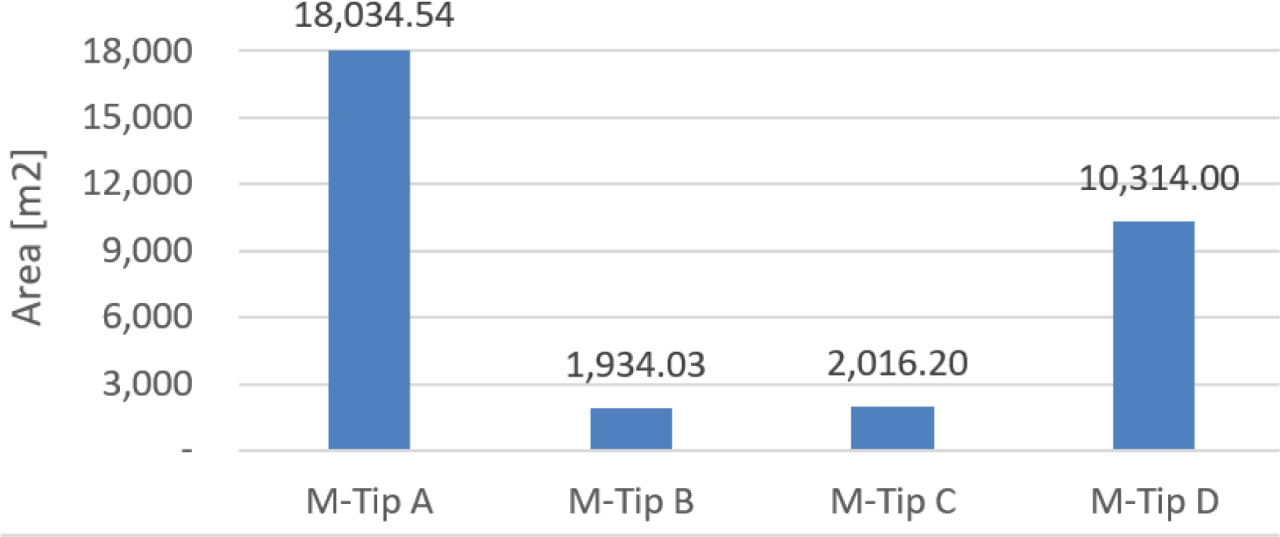
According to the Kosovo Agency of Statistics [43], the population number in Kosovo in 2011 was 1,739,825 residents, whereas the mean used area per capita is 13.3 m2. This means that Kosovo had 23,139,672 m2 of residential area in 2011. On the other hand, according to the growth estimation statistics from [44] and [45], Kosovo in 2020 had 1,783,531 residents and according to [26] it has 32,298,777 m2 residential area, meaning that the growth in the residential area from 2011-2017 can be inferred to an estimate of 9,159,105 m2. Additionally, the mean of used area per capita has increased to 18,11m2. This shows that a continuous increase in electricity and heating demand is inevitable.
In the following section, the yielded results are presented and discussed regarding the heat load, the financial analysis, and the environmental impact.
The results of retrofit after applying EEM are presented and compared with the base reference model. Figure 13 shows that the thermal energy consumption for a heating season of the reference building before EEM (Scenario S-01/NE-EB), namely the existing conditions, is 193.27 MWh/year when adding all heat loads for walls, windows, roof, and basement. Then, the total heat load is compared to S-02/EE-EB, S-03/EE-HP and S-04/EE-DH which is 87.69 MWh/year. Meaning that the thermal energy consumption is higher in the current state for 105.62 MWh/year or 220.45%.
Heating load for the reference building envelope (wall, window, roof, and floor)
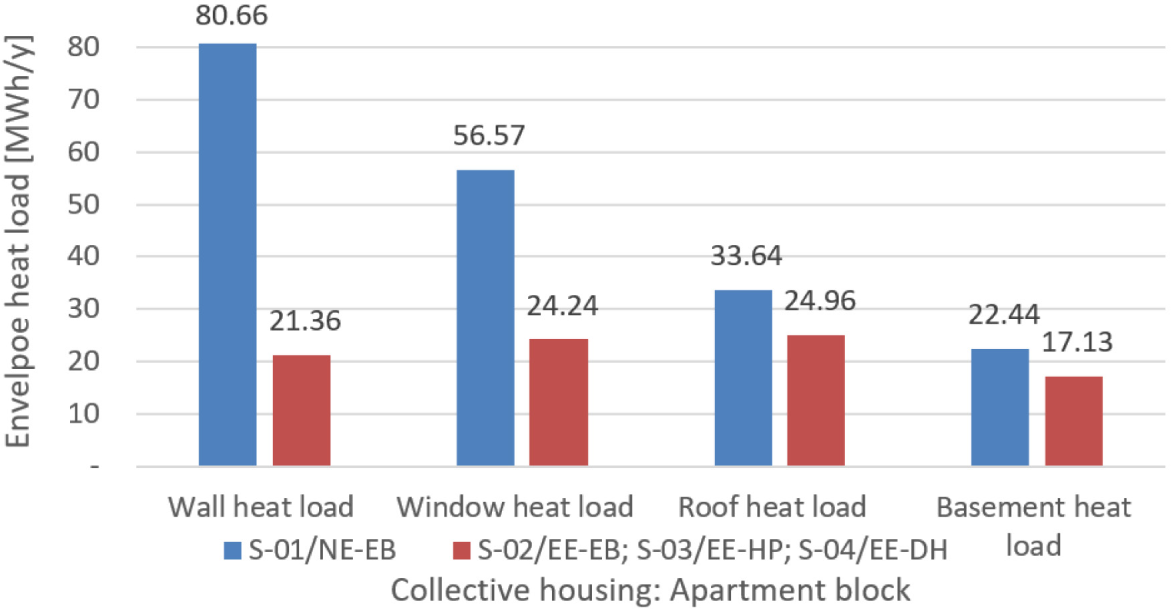
The significant thermal losses are attributed to the envelope of the reference AB. The heating systems power was calculated according to the thermal losses. The results depicted in Figure 14 shows that the calculated system power for S-01/NE-EB resulted in 251.44 kW, which is 109.83 kW higher or 177.56%, compared to scenarios S-02/EE-EB, S-03/EE-HP and S-04/EE-DH which is 141.61 kW.
Figure 14 also presents the energy consumption for different scenarios. The yielded results show that scenario S-03/EE-HP using heat pumps has low energy consumption, considering air-water heat pump with COP=2.89.
Figure 15 shows that the specific heat input for S-01/NE-EB is qh=174.15 kWh/m2/year; for scenario S-02/EE-EB, S-03/EE-HP and S-04/EE-DH it is the same, qh=79.66 kWh/m2/year. The lowest specific fuel input is encountered in S-03/EE-HP that is qf=27.57 kWh/m2/year.
Calculated heating and fuel loads for the reference AB building model for different scenarios. The heating load is significantly reduced after the application of EEM and the lowest fuel consumption is in scenario 3 for heat pumps
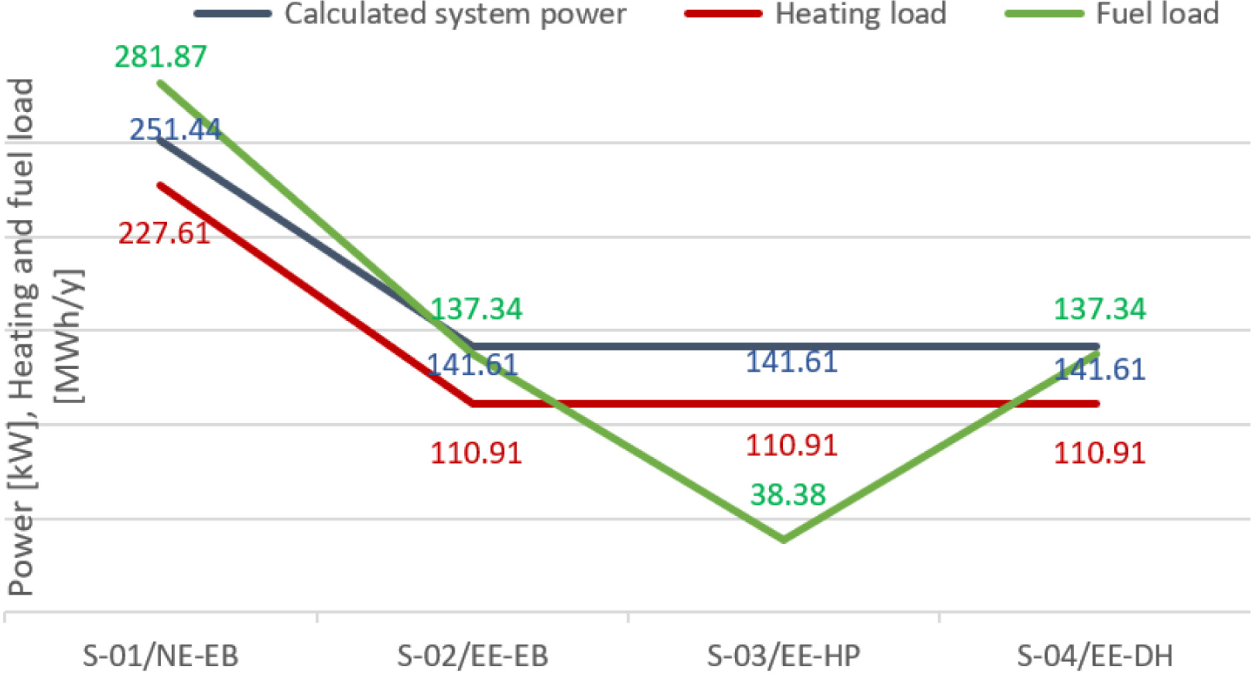
Specific heat and fuel inputs for the reference AB building model for different scenarios. The lowest fuel consumption annually per m2 is in scenario 3 for heat pumps
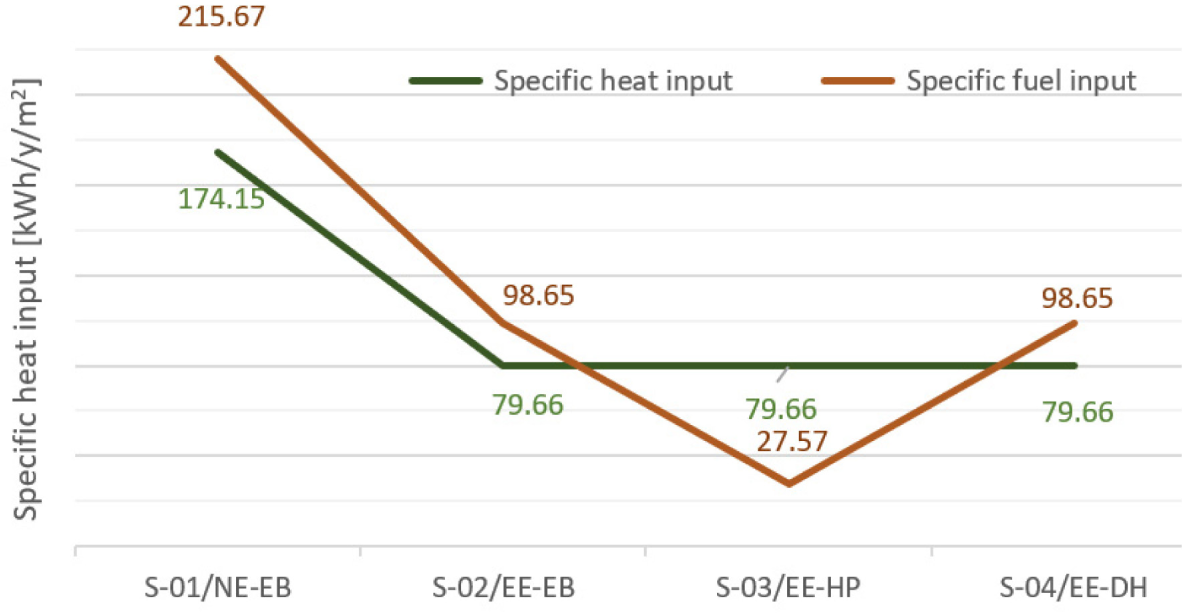
The use of EEM in a building and the selection of heating systems have a significant impact on the financial aspect as well. Therefore, it is important to compare the performance of each scenario in terms of financial implications.
The impact of the proposed EEM in different scenarios fuel cost and savings are presented in Figure 16, compared to the current state of the reference building.
The results show that the heating cost in scenario S-01/NE-EB presenting the existing state that has no EEM and uses electric boilers is 20,548 Euro/year, when adding EEM to the building with the same heating system (S-02/EE-EB) the cost drops to 10,013 Euro/year resulting in monetary savings of 10,535 Euro/year. Furthermore, if the heating system is substituted with heat pumps (S-03/EE-HP), the cost savings reach a maximum of 17,750 Euro/year compared to the cost savings using district heating (S-04/EE-DH) which is 13,681 Euro/year.
Total fuel cost and fuel savings for the reference AB building model
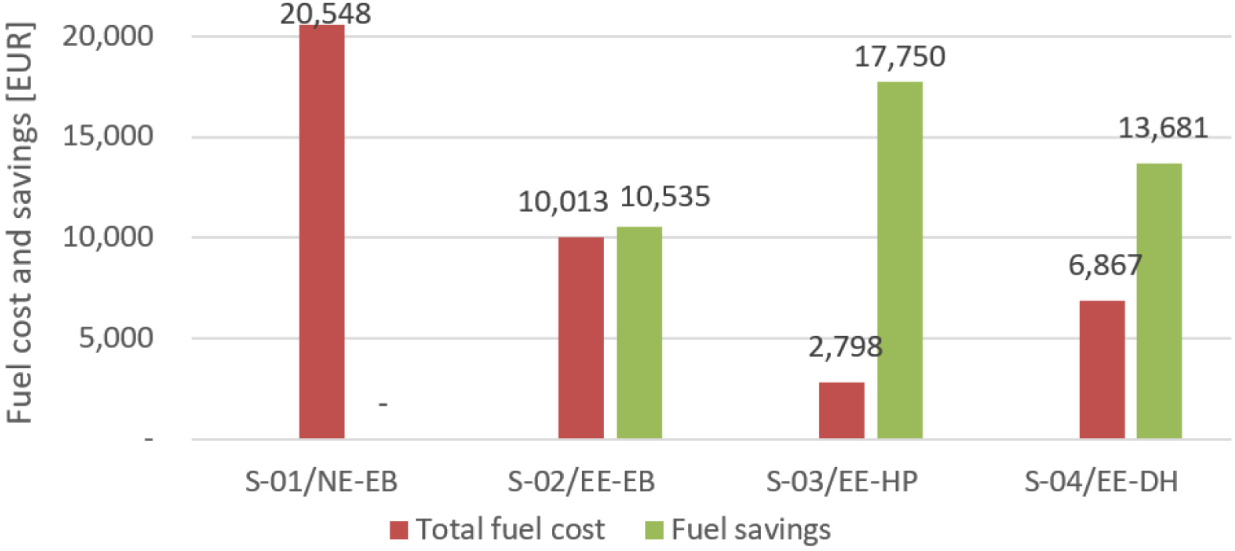
When comparing the electricity, thermal and fuel costs on one side, and the carbon emissions on the other, also by estimating the cost of an electric boiler with a capacity of 12-24 kW by 750 Euro/unit and the cost of a 13.7 kW heat pump by 7,200 Euro/unit, two conclusions can be drawn: 1) The specific cost per kWh for heating from electric energy is 0.073 €c/kWh, which is 209% higher than heating with wood as fuel, and 143% higher than the pellet as presented in Figure 17. The capacities of the electric boiler and heat pumps have been evaluated according to the heating demand. However, since in most AB there are no storage areas for fuel, as well as the high price of wood or pellet boilers, it makes the electricity a more convenient solution for heating although for a higher cost. 2) When considering heat pumps as a HVAC solution, their initial price is 960% higher than electric boilers, but the energy saving per residential unit is 171.78 Euro/year, meaning that the payback period is 37.68 years. This payback is attributed to the high initial investment but it goes in line with the range reported in an economic analysis for heat pumps in research [46] which starts from 28 years and can go up to 34 years depending on other factors as well such as climate conditions [47], energy prices and energy use, etc.
Comparison of the impact of fuel type on environmental impact and costs
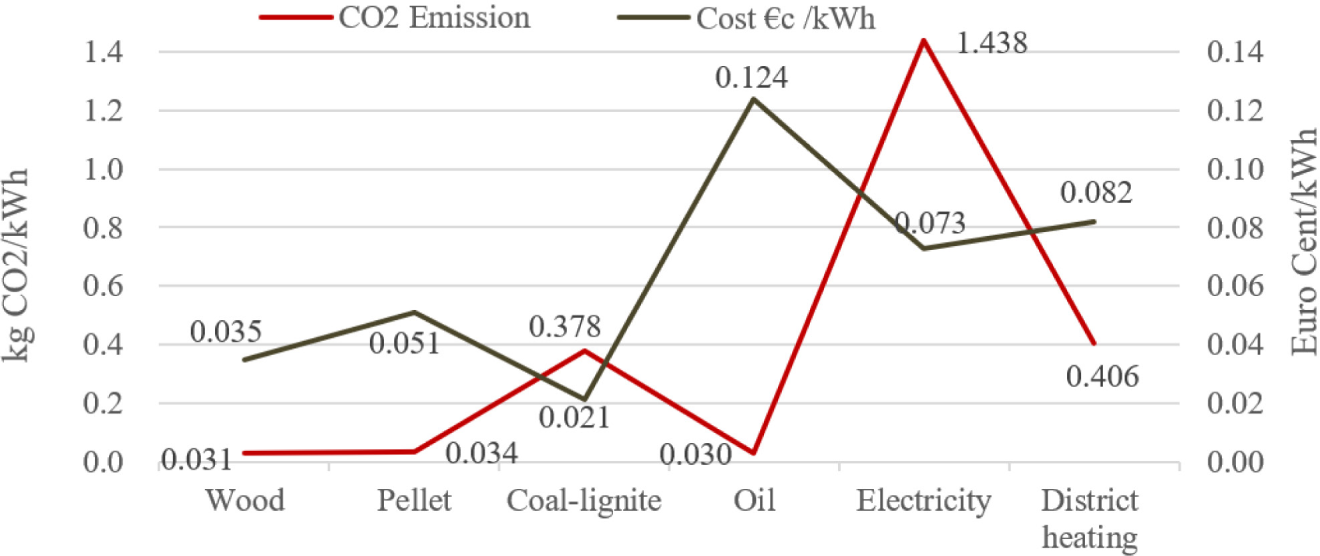
The high energy demand also results in the high environmental impact of the existing reference AB building. Figure 18a shows the annual CO2 emissions based on energy and fuel consumption. The fuel savings facilitate the estimation of the CO2 emission mitigation of saving that is presented in Figure 18b. In line with the energy consumption, the highest energy consumer (S-01/NE-EB) has also the highest CO2 emission level of 405,329 kgCO2/year" , followed by S-02/EE-EB with 197,495 kgCO2/year, S-03/EE-HP with 55,190 kgCO2/year and lastly S-04/EE-DH with 55,760 kgCO2/year. Thus, when it comes to environmental impact, the best scenarios are S-03/EE-HP and S-04/EE-DH, resulting in CO2 savings of 86.38% and 86.24%, respectively for one building. The potential of reducing CO2 complies with the findings of [20] where they achieved 49.7% up to 68.3% CO2 emission reduction on an urban level for the same city for different building typologies. However, compared to these values, the benefits from retrofitting in terms of CO2 savings can reach up to 95%, as stated in [48]. Furthermore, the value can be further improved by introducing renewable energy technologies, equipping the building with energy management systems, or applying more green measures such as green roofs [49] and increasing the energy awareness of the occupant behaviour [50].
Collective housing – block apartment a) CO2 emission and b) CO2 mitigation for different scenarios per year.
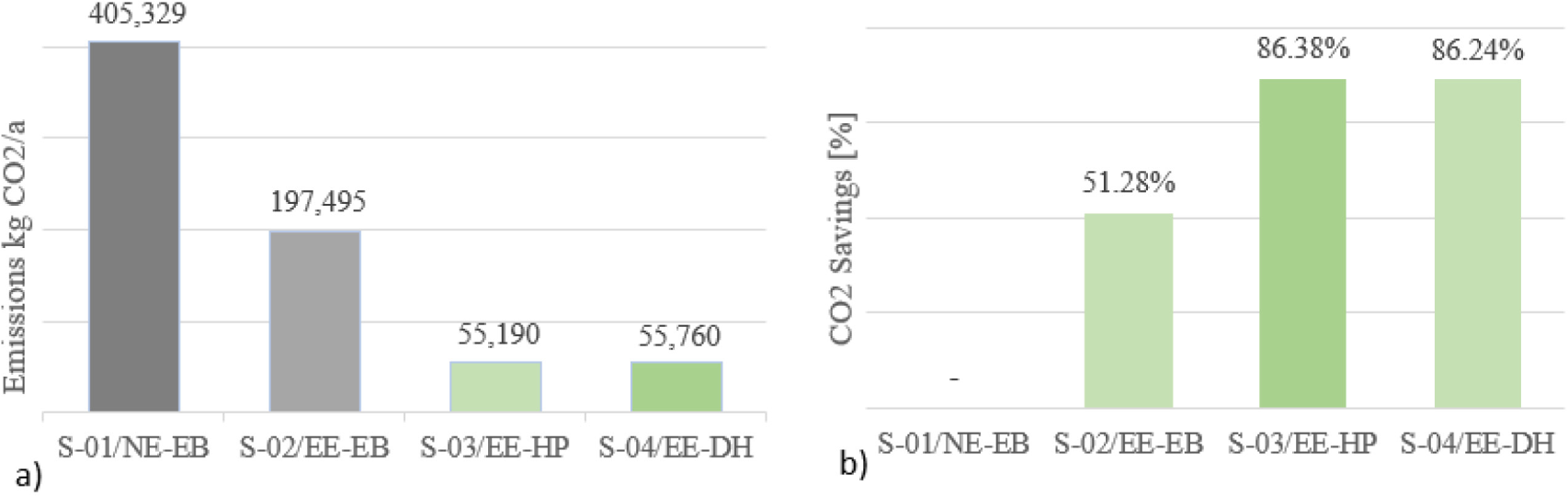
Therefore, based on these findings and based on Law no. 06/L-079 [51] on energy efficiency it is recommended to accelerate the implementation of EEM in existing buildings, especially older buildings, to decrease the heating energy consumption, costs and environmental impact. Furthermore, the proper selection of the HVAC systems is of high importance in building energy performance. The application of such measures apart from having a positive influence on the energy sector also affects the economic sector indirectly by increasing the production of energy-efficient materials such as thermal insulations, doors and windows which also influences the employment rate.
This study presents the implementation of the existing regulations of building energy performance such as Regulation MESP-04/2018-RR [10] and MESP -02/2018-RR [11] to evaluate the impact on the energy demand, costs, and environmental impact of existing AB. The evaluation and comparison of the existing state to different scenarios are presented, where various EEM and heating solutions combinations are discussed. The results show that only by retrofitting the building envelope including thermal insulation of walls, roof, and floors, and by changing windows according to the regulation directives, an increase in energy-saving is achieved by 378% (59.30MW/year) from the walls and 233% (32.33MWh/year) from windows alone. The heating load is reduced by 116.7 MWh/year and the fuel demand reduction by 243.49 kWh/year according to scenario S-03/EE-HP with heat pumps. From the financial perspective, solely by applying simple EEM cost savings of up to 10,535 Euro/year can be reached. Furthermore, if district heating is used instead of electric boilers the cost savings reach 13,681 Euro/year. Lastly, if heat pumps are installed, then the AB reference building reaches cost savings up to 17,750 Euro/year. Looking from the environmental lens, the applied EEM and HVAC system facilitate 86% mitigation of CO2 emissions of the reference AB when compared to the existing state of the building. If these measures are applied to the other 19 similarly constructed AB buildings in Prishtina, which consist of 798 residential units, then it would be very beneficial to the energy savings, costs, and environmental impact, and it would alleviate the temporal and spatial mismatch of energy supply and demand. Therefore, the findings of this research are important as they emphasize the impact and promote the enforcement of existing regulations regarding building energy performance to the building sector stakeholders.
Ai |
surface are of the building envelope |
[m2] |
Bfuel |
fuel load |
[MWh/a] |
CO2sp |
specific CO2 emission of fuel type |
[kgCO2/kWh] |
EMCO2 |
CO2 emission annually |
[kgCO2/a] |
f |
conversion factor from Wh to MJ |
[-] |
HT,k |
transfer coefficient by transmission to adjacent spaces |
[W/K] |
LHV |
lower heating value of the fuel |
[kJ/kg] |
lk |
length of linear thermal bridge |
[m] |
t |
duration of calculation period e.g., month |
[-] |
Ui |
thermal transmission coefficient (U-value) |
[W/(m2K)] |
QN,H |
building energy demand for heating |
[MJ] |
QL,H |
total heat transfer (losses) for heating mode |
[MJ] |
QG,H |
total heat sources (gains) for heating mode |
[MJ] |
QL |
total heat transfer |
[MJ] |
QT |
Total heat transfer by transmission |
[MJ] |
QV |
total heat transfer by ventilation |
[MJ] |
Qi |
sum of internal heat sources |
[MJ] |
Qs |
sum of solar heat sources |
[MJ] |
Qsun,t |
solar heat gain through transparent surfaces |
[MJ] |
Qsun,nt |
solar heat gain through the opaque surfaces |
[MJ] |
Qi,occ |
internal heat production from occupants |
[MJ] |
Qi, app |
internal heat production from appliances |
[MJ] |
t |
duration of calculation period e.g., month |
- |
Ui |
thermal transmission coefficient (U-value) |
[W/(m2K)] |
Greek letters |
||
ηG,H |
the utilization factor of heating gains. |
[-] |
θi |
internal temperature of the building zone |
[°C] |
θe,k |
external temperature |
[°C] |
Ψk |
thermal conductivity |
[W/(mK)] |
Abbreviations |
||
AB |
Apartment Blocks |
|
CH |
Collective Housing |
|
CPP |
Coal-fired Power Plant |
|
DH |
District Heating |
|
EB |
Electric Boiler |
|
EE |
Energy Efficiency |
|
EEM |
Energy Efficiency Measures |
|
HP |
Heat Pump |
|
NE |
No Energy Efficiency Measures |
|
MESP |
Ministry of Environment and Spatial Planning |
|
MESTI |
Ministry of Education, Science, Technology and Innovation |
|
PP |
Power Plant |
Energy retrofit of historic and existing buildings. The legislative and regulatory point of view ,Energy and Buildings , Vol. 95 ,pp 23–31 , , https://doi.org/https://doi.org/10.1016/j.enbuild.2014.10.073
, - Climate Change: Implications for Buildings. Key Findings from the Intergovernmental Panel on Climate Change Fifth Assessment Report commissioned by BPIE, G BPN, WBCSD, CJBS ECF, 2014, http://bpie.eu/publication/climate-change-implications-for-buildings
- , Technical Summary. In: Climate Change 2022: Mitigation of Climate Change. Contribution of Working Group III to the Sixth Assessment Report of the Intergovernmental Panel on Climate Change, 2022
- , European Parliament, Directive 2002/91/EC of The European Parliament and of the Council of 16 December 2002 on the energy performance of buildings, L 1/65,2003 04.1
- , European Parliament, Directive 2010/31/EU of The European Parliament and of the Council of 19 May 2010 on the energy performance of buildings (recast), L 153/13, 18.6.2010
Policy strategies for achieving large long-term savings from retrofitting existing buildings ,Energy Efficiency , Vol. 12 (1),pp 89–105 , , https://doi.org/https://doi.org/10.1007/s12053-018-9661-5
, Building Regulations Related to Energy and Water in Indian Hill Towns ,J. sustain. dev. energy water environ. syst. , Vol. 5 (4),pp 496-508 , , https://doi.org/https://doi.org/10.13044/j.sdewes.d5.0161
, Non-Compliance with Building Energy Regulations: The Profile, Issues, and Implications on Practice and Policy in England and Wales ,J. sustain. dev. energy water environ. syst. , Vol. 1 (4),pp 340-351 , , https://doi.org/https://doi.org/10.13044/j.sdewes.2013.01.0026
, Analysis of the use of different standards for estimation of energy efficiency measures in the building sector ,J. sustain. dev. energy water environ. syst. , Vol. 10 (1),pp 1-16 , , https://doi.org/https://doi.org/10.13044/j.sdewes.d8.0375
, - Regulation No. 04/18 For minimum requirements for the energy performance of buildings, 2018, https://gzk.rks-gov.net/ActDocumentDetail.aspx?ActID=18297
- REGULATION (MESP) No. 02/18 on national calculation methodology for integrated energy performance of buildings, 2018, https://gzk.rks-gov.net/ActDocumentDetail.aspx?ActID=18295
Model-Based Comparative Evaluation of Building and District Control-Oriented Energy Retrofit Scenarios ,Buildings , Vol. 8 (7), , https://doi.org/https://doi.org/10.3390/buildings8070091
, Energy and Economic Assessment of Energy Efficiency Options for Energy Districts: Case Studies in Italy and Egypt ,Energies , Vol. 14 (4), , https://doi.org/https://doi.org/10.3390/en14041012
, Energy-saving potential and cost-effectiveness of active energy-efficiency measures for residential building in warm-humid climate ,Energy for Sustainable Development , Vol. 67 ,pp 163–176 , , https://doi.org/https://doi.org/10.1016/j.esd.2022.01.011
, Environmental and Economic Analysis of Heating Solutions for Rural Residences in China ,Sustainability , Vol. 14 (9), , https://doi.org/https://doi.org/10.3390/su14095117
, Life-cycle approach to the estimation of energy efficiency measures in the buildings sector ,Applied Energy , Vol. 264 ,pp 114745 , , https://doi.org/https://doi.org/10.1016/j.apenergy.2020.114745
, Retrofitting Existing Buildings to Improve Energy Performance ,Sustainability , Vol. 14 (2), , https://doi.org/https://doi.org/10.3390/su14020666
, Application of multi-objective optimization model to assess the energy efficiency measures for the cases of Spain ,Journal of Building Engineering , Vol. 38 ,pp 102144 , , https://doi.org/https://doi.org/10.1016/j.jobe.2020.102144
, Assessment of energy efficiency measures using multi-objective optimization in Portuguese households ,Sustainable Cities and Society , Vol. 35 ,pp 764–773 , , https://doi.org/https://doi.org/10.1016/j.scs.2017.09.032
, A novel spatial based approach for estimation of space heating demand saving potential and CO2 emissions reduction in urban areas ,Energy , Vol. 225 ,pp 120251 , , https://doi.org/https://doi.org/10.1016/j.energy.2021.120251
, Analysis of the Potential for Renewable Utilization in Kosovo Power Sector ,Environments , Vol. 7 (6), , https://doi.org/https://doi.org/10.3390/environments7060049
, Increasing the integration of variable renewable energy in coal-based energy system using power to heat technologies: The case of Kosovo ,Energy , Vol. 212 ,pp 118762 , , https://doi.org/https://doi.org/10.1016/j.energy.2020.118762
, - , Current and future emissions of GHG gases and air pollutants from coal-fired power plants in Kosovo, in Proceedings of the 2nd SDEWES SEE Conference, 2016
An exergy-rational district energy model for 100% renewable cities with distance limitations ,Therm sci , Vol. 24 (6),pp 3685–3705 , 2020, https://doi.org/https://doi.org/10.2298/TSCI200412287K
, Design Optimization of Energy Efficient Residential Buildings in Mediterranean Region ,J. sustain. dev. energy water environ. syst. , Vol. 10 (2),pp 1-21 , , https://doi.org/https://doi.org/10.13044/j.sdewes.d9.0385
, - Guidelines for implementation of minimum requirements for energy performance in Existing buildings in the residential sector - Typology of buildings in the republic of Kosovo, 2019, https://konsultimet.rks-gov.net/Storage/Consultations/13-10-03-15032019/1.%20Tipologjia%20pjesa%20e%20pare.pdf
- Termokos – Ngrohtorja e qytetit, https://termokos.org/en/home-3
- Diesel costs, https://www.kosovo.shell.com
- Electricity Tariffs, https://www.ero-ks.org/zrre/sq/konsumatoret-shtepiak/energjia-elektrike/tarifat-e-energjise-elektrike
- District Heating Tariffs, https://www.ero-ks.org/zrre/en/konsumatoret-shtepiak/energjia-termike/tarifat-e-energjise-termike
- Annual Report 2020, https://www.ero-ks.org/zrre/sites/default/files/Publikimet/Raportet%20Vjetor/Raporti%20vjetor%202020_ZRRE_shqip.pdf
- Annual balance report for electricity and thermal energy for 2020, https://www.ero-ks.org/zrre/sites/default/files/Publikimet/Raportet%20Vjetor/Raporti%20vjetor%202020_ZRRE_shqip.pdf
- Electricity Cost Calculator, https://www.ero-ks.org/zrre/sq/konsumatoret-shtepiak/energjia-elektrike/kalkulatori
- Tarifat dhe cmimet e miratuara nga ERE per vitin 2020, https://ere.gov.al/doc/Tarifat_dhe_Cmimet_ne%20_fuqi_per_vitin_2020.pdf
- Household Consumption Calculator, http://kalkulator.eps-snabdevanje.rs/kalkulator
- Calculate your bill based on registered consumption, https://www.epcg.com/domacinstva/kalkulacija-po-tarifnim-modelima
- , https://www.evn.mk
- Calculator, https://www.epbih.ba/stranica/invoices-and-payment#calculator
- Information about the Cogeneration Project, 2017, https://termokos.org/en/2017/09/21/1994/
Zero carbon energy system of South East Europe in 2050 ,Applied Energy , Vol. 184 ,pp 1517–1528 , , https://doi.org/https://doi.org/10.1016/j.apenergy.2016.03.046
, - , Europe’s buildings under the microscope. A country-by-country review of the energy performance of buildings, Buildings Performance Institute Europe
- EU Allocates Additional Grant to introduce District Heating in Eight Municipalities in Kosovo, https://wbif.eu/news-details/eu-allocates-additional-grant-introduce-district-heating-eight-municipalities-kosovo
- Kosovo Agency of Statistics, https://ask.rks-gov.net/en/kosovo-agency-of-statistics/general-statistics
- Country Meters - Population growth in Kosovo, https://countrymeters.info/fr/Kosovo
- Estimation of Kosovo population 2017, https://ask.rks-gov.net/media/4174/estimation-kosovo-population-2017.pdf
Analysis of thermal performance and economy of ground source heat pump system: a case study of the large building ,Geothermics , Vol. 89 ,pp 101929 , , https://doi.org/https://doi.org/10.1016/j.geothermics.2020.101929
, Techno-economic review of solar heat pump systems for residential heating applications ,Renewable and Sustainable Energy Reviews , Vol. 81 ,pp 22–32 , , https://doi.org/https://doi.org/10.1016/j.rser.2017.07.041
, Strategies and scenarios to reduce energy consumption and CO2 emission in the urban, rural and sustainable neighbourhoods ,Sustainable Cities and Society , Vol. 72 ,pp 103053 , , https://doi.org/https://doi.org/10.1016/j.scs.2021.103053
, Costs and Benefits of Green Roof Types for Cities and Building Owners ,J. sustain. dev. energy water environ. syst. , Vol. 7 (1),pp 57-71 , , https://doi.org/https://doi.org/10.13044/j.sdewes.d6.0225
, - , Occupant preferences on the interaction with human-centered control systems in school buildings, Journal of Building Engineering
- Law No. 06/L-079 On Energy Efficiency, 2018, https://gzk.rks-gov.net/ActDetail.aspx?ActID=18216